Chromatographic Purification of Plasmid DNA (pDNA) for mRNA, Plasmid-based DNA Vaccines, and Viral Vector Applications
Section Overview
- Role of Chromatography in Plasmid DNA Production
- Types of Chromatography Available for Plasmid DNA Production
- High Salt Concentrations Maximizes pDNA Capture
- pDNA Purification Challenges with Conventional Chromatography Resins
- High-throughput Capture Using Anion Exchange Chromatography
- Case Study 1: Increasing Salt Concentration Improves pDNA Purity During Anion Exchange (AEX) Chromatography by Removing Contaminating RNAs
- Case Study 2: Anion Exchange Wash Optimization
- Case Study 3: Anion Exchange Productivity Comparison: Resin vs. Membrane
- Related Products
Widespread application of pDNA in vaccines and gene therapy is driving increased demand and as a response, plasmid manufacturing must become more efficient with improved productivity. Intensification of chromatographic steps can help address this demand.
Role of chromatography in plasmid DNA production
The purification of pDNA is difficult because many of the critical impurities are negatively charged, similar in size, and possess similar levels of hydrophobicity. The final bulk pDNA must meet quality specifications set by regulatory agencies for purity and must contain greater than 90% pDNA in a supercoiled isoform. Chromatography steps are used to purify pDNA so that it meets these regulatory standards. It is used both as a process step to achieve greater purity and as an analytical tool to monitor pDNA quality.
Types of chromatography available for plasmid DNA production
The most commonly used techniques for plasmid purification are anion exchange chromatography (AEC) and hydrophobic interaction chromatography (HIC). Both techniques have been implemented for capture or intermediate purification/polishing and are often combined.1,2 Size exclusion chromatography (SEC) is sometimes included as part of the downstream scheme; it is typically chosen as the last step due to its disadvantages of low throughput and slow kinetics.1,3 HIC is able to separate the native supercoiled pDNA from pDNA isoforms from more hydrophobic nucleic acid impurities (RNA, genomic DNA, denatured pDNA) and from endotoxins.4 AEC achieves the removal of proteins, low molecular weight RNA, and endotoxins but its efficiency is highly dependent on sample composition.
High Salt Concentrations Maximizes pDNA Capture
A high salt concentration in the load should be applied to maximize pDNA capture.1,5
To demonstrate this, we used a standard feed as starting material for purification runs from original E. coli lysate,3 clarified by centrifugation and subsequent depth filtration, and directly supplemented with NaCl.
Salt supplementation of the clarified feed prior to anion exchange capture chromatography is a particularly useful approach for removing RNA impurities without requiring RNase. The RNA species bind less strongly to anion exchange media as compared to the more strongly charged pDNA. Therefore, salt supplementation to an optimal conductivity will allow RNA impurities to flow through the AEX adsorbent unbound, while pDNA binds and is subsequently eluted with high purity.
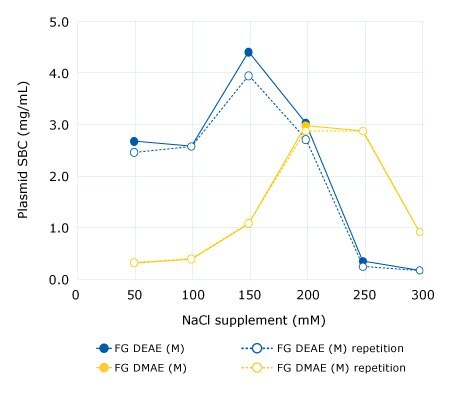
Figure 1.Batch assay for determination of optimal NaCl concentration for lysate supplementation. Static binding capacities (SBC) were measured in 96-well filter plates (1 mL per well). Plasmid feed was original clarified lysate (pH 5.0, 67 mS/cm) supplemented with increasing NaCl concentrations. FG = Fractogel® EMD resin.
The optimal salt concentration for supplementation must be pre-determined prior to the purification runs for each resin/membrane adsorber. This is done by measuring plasmid binding capacity at increasing sodium chloride concentrations. The principle is demonstrated with the examples of Fractogel® EMD DEAE (M) and Fractogel® EMD DMAE (M) resins in Figure 1. In Case Study 1, we also demonstrate how salt concentrations affect capacity, purity, and recovery of pDNA.
pDNA Purification Challenges with Conventional Chromatography Resins
For conventional chromatography resins, pDNA purification is a challenge because it is much larger than the proteins for which resins were originally designed. pDNA’s size prevents it from entering the pore structure of resin beads causing low binding capacity and slow mass transfer.4,5 Additionally, the (larger) size increased pressure drop and processing time due to feed viscosity, low resolution of isoforms, and potential fouling. Despite the drawbacks, most existing large-scale process utilize chromatography resins because of their comparatively high resolution.
However, pDNA manufacturers are undertaking an intensification of chromatography steps using convective media (i.e. membranes, monoliths, and fiber-based technologies) to improve productivity and increase overall output.
High-throughput capture using anion exchange chromatography
As high-throughput capture using anion exchange chromatography is widely used in plasmid DNA production, we performed several optimization studies using our Natrix® Q chromatography membranes.
Natrix® Q chromatography membrane devices provide significant performance improvement compared to traditional resins. Productivity (g/L/hr) increases dramatically because convective channels created by the macroporous polymer structure have a high-density of quaternary amine binding sites with rapid mass transfer. These binding sites are accessible to even very large target molecules, like pDNA, at fast flow rates while maintaining purification targets required for GMP manufacturing. With a primary capture binding capacity of ~10 mg/mL from a clarified lysate supplemented with NaCl, Natrix® Q chromatography membrane can remove greater than 95% of the initial large RNA. In one example, Natrix® Q chromatography membrane achieved ≥80% pure plasmid DNA, with ~10% residual RNA (A260 based), and a yield of ~80% with only 30 minutes of runtime.
Case Study 1: Increasing Salt Concentration Improves pDNA Purity During Anion Exchange (AEX) Chromatography by Removing Contaminating RNAs
Objective
Optimize NaCl concentration in the load material to promote capture of plasmid DNA product while RNA impurities are removed in the load flowthrough.
Materials
- Device - Natrix® Q Micro 0.2 mL. A new Natrix® Q device was used for each test.
Clarified Lysate Conditions
- 6.5 kbp pDNA, 24 µg/mL titer. 1.5 M K-acetate buffer, pH 5.3, 86.9 ms/cm
- Nucleic acid content: 3.8% pDNA, 96.2% RNA. Endotoxin content: 380,000 EU/mg pDNA
- The clarified lysate was loaded to 11 mg pDNA/mL of membrane at three different load conductivities.
- Test 1: No additional salt was added.
- Test 2: 35 mM NaCl was supplemented into the clarified lysate
- Test 3: 75mM NaCl was supplemented into the clarified lysate
Analytics
- DNA and RNA content assessed by HPLC (Tosoh DNA-NPR method)
- Endotoxin content assessed by Charles River Endosafe assay
Results
A high binding capacity at 11 mg of plasmid per mL of membrane was achieved when no sodium chloride was added into the feed. However, the eluate plasmid DNA purity was quite low for this condition – just 46% of eluate nucleic acid was pDNA, with the remainder being RNA. This indicates that a high percentage of RNA bound to the membrane under these load conditions.
When the salt concentration was increased to 35 mM, there was a slight reduction in binding capacity to 8 mg plasmid per mL of membrane. However, most of the RNA impurity flowed through the membrane at this elevated conductivity, resulting in improved purity (77% of eluate nucleic acid was pDNA). High yield was also observed in the elution pool at 88%. Endotoxin clearance was also achieved with this strategy; the elution pool has 3,100 endotoxin units per mg of plasmid.
When the NaCl concentration was further increased to 75 mM, a significant drop-off in binding capacity was observed. At this elevated concentration neither the RNA nor the plasmid DNA product are able to sufficiently bind to the anion exchange membrane (Figure 2). It was therefore determined that lysate supplementation with 35 mM NaCl offered the best balance of capacity, purity, and recovery on Natrix® Q membrane.
Salt supplementation of the clarified feed prior to anion exchange capture chromatography is a particularly useful approach for removing RNA impurities without requiring RNase. The RNA species bind less strongly to anion exchange media as compared to the more strongly charged pDNA. Therefore, salt supplementation to an optimal conductivity will allow RNA impurities to flow through the AEX adsorbent unbound, while pDNA binds and is subsequently eluted with high purity.
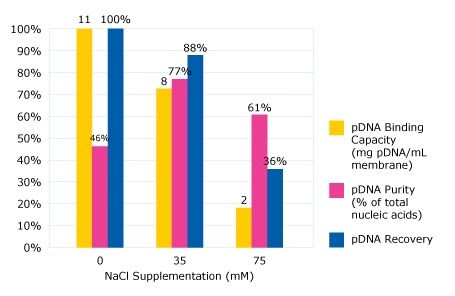
Figure 2.Impact of salt supplementation on capacity, purity, recovery of pDNA.
Case Study 2: Anion Exchange Wash Optimization
Objective
Assess the ability of detergent and EDTA wash buffers to further improve Natrix® Q RNA and endotoxin clearance.
Materials
- Device - Natrix® Q Micro 0.2 mL. A new Natrix® Q device was used for each test.
- Feed - Clarified and sterile filtered pDNA lysate, supplemented with 35 mM NaCl
To optimize the wash strategy for the anion exchange capture step, control wash conditions were compared to an experimental detergent wash strategy where a low concentration of detergent was added to the wash as well as 2 mM EDTA to a wash and elution buffer. This approach improved the nucleic acid purity from 77% to 95% and reduced the endotoxin concentration to 500 endotoxin units per milligram of plasmid.
Case Study 3: Anion Exchange Productivity Comparison: Resin vs. Membrane
Objective
Compare the observed productivity of Natrix® membrane chromatography against historical reference data for a chromatography resin.
Materials
- Adsorbers - Performance of Natrix® Q chromatography membrane compared to historical reference data for Fractogel® DMAE resin data (see to Table 6 for resin chromatography reference data)
- Feed basis - Consider a 300 L batch of clarified lysate containing 7.1 g pDNA
Results
Use of Natrix® Q chromatography membrane increased productivity of the chromatography step 24x, from 0.30 g pDNA/L/hr to 7.3 g pDNA/L/hr. These gains can be attributed to two factors:
- The binding capacity of pDNA for the membrane is 8 g/L compared to just 3 g/L for the traditional resin; and
- Membrane chromatography offers very fast flowrates due to its open pore structure, enabling a cycle time of just over 1 hour while the slower resin process can require nearly 10 hours per cycle. This can be leveraged to run several cycles with membrane chromatography in a rapid cycling approach.
Learn more about the pDNA manufacturing process in our webinar “Effective and Efficient Design of a Downstream Purification Process for Plasmid DNA.”
Selecting chromatography products and methods can be a challenge, as they are highly process-specific and often play a critical role at multiple steps from early to late downstream. To simplify this challenge for biopharmaceutical manufacturers, learn more about our Emprove® Chromatography portfolio, built to address specific needs for streamlined resin selection and qualification.
References
続きを確認するには、ログインするか、新規登録が必要です。
アカウントをお持ちではありませんか?