Overcoming Aggregation in Solid-phase Peptide Synthesis
The 3D structure, and consequently bioactivity, of individual proteins is largely determined by the primary sequence of its component amino acids. This inherent propensity of peptide chains to form aggregated structures is the principle cause of the highly sequence specific variability in synthetic efficiency encountered during peptide assembly. As the peptide is elongated, it can form secondary structures that cause peptide chain aggregation, where individual peptide molecules come together to form larger, often insoluble structures or aggregates, resulting in lower reaction rates. The effects can range from just a subtle slowing down to a complete failure of both deprotection and acylation reactions. In such extreme cases, the peptide has effectively become insoluble and is no longer available for reaction.
The onset of severe aggregation in batch synthesis is generally indicated by shrinking of the resin matrix, whereas in continuous flow synthesis, it is detected by flattening and broadening of the deprotection profile. In such circumstances usual coupling tests with ninhydrin or TNBS are often no longer reliable and can give false negative results.
The ease of assembly of a given sequence is generally hard to predict and is one of the factors that makes peptide synthesis interesting and challenging. Peptides containing stretches of contiguous hydrophobic amino acids like Ala, Val, Ile as well as those containing amino acids, which can form intra-chain hydrogen bonds, such as Gln, Ser and Thr, are frequently difficult to make. For this reason, it is generally better to adopt from the outset synthetic strategies that mitigate the effects of structure formation, rather than trying to second guess which peptides may be problematic and wasting time and resources repeating failed syntheses. For peptide sequences longer than 20 amino acids it is therefore strongly recommended to monitor the peptide assembly by small TFA cleavages.
The majority of approaches developed to ameliorate this issue attempt to improve the solvation of the peptide-resin complex. These include using dipolar aprotic solvents, such as DMF, DMSO or NMP, chaotopic salts, special solvent cocktails like “Magic Mixture”, or PEG-based polar resins like NovaSyn® TG, NovaPEG or PEGA (Table 1). The degree of cross-linking of the polystyrene resin with DVB can also play a key role and should not be higher than 1%, otherwise proper swelling can be inhibited. Resin functionalization is another important factor, with high loading resins exacerbating the effects of aggregation, and it is for this reason that the Novabiochem® product line offers special low-loading versions of the most popular supports used for Fmoc SPPS. The choice of side-chain protecting group can also influence peptide-solvation. Substitution of Ser(tBu) or Thr(tBu) with the Trt derivatives, or Lys(Boc) with Lys(Tfa) can have a beneficial effect. However, the most universally effective way of improving synthetic efficiency is to reversibly protect the amide backbone of key residues through the introduction of secondary amino acid surrogates
For a detailed discussion on how to identify and overcome the effects of aggregation, the reader is referred to the excellent article by Quibell & Johnson.10
Secondary Amino Acid Surrogates
Secondary amino acid surrogates are analogs of proline or N-alkylamino acids that are derived from standard primary amino acids by reversible protection of the backbone amide bond. They work by mimicking the natural propensity of proline and N-alkyl amino acids to disrupt the formation of the secondary structures during peptide assembly. Their use leads to better and more predictable acylation and deprotection kinetics, which results in higher purities and solubilities of crude products, easier HPLC purification and improved yields, with less need to repeat failed syntheses. They have proved particularly effective in the synthesis of intractable peptides, long peptides/small proteins, and cyclic peptides, enabling in many cases the production of peptides that otherwise could not be made.
Novabiochem at present supplies three kinds of secondary amino acid surrogates: pseudoproline dipeptides; Dmb dipeptides; Dmb/Hmb amino acids (Figure 1). All work on the same principle by temporarily introducing a structure breaking amino acid into the peptide sequence. The effects can be long range, with formation of structures often being postponed for as many as six residues, or eliminated altogether. Following peptide assembly, treatment with TFA cleaves the amide bond protecting group regenerating the native sequence containing the primary amino acid from which the secondary amino acid surrogate was derived.
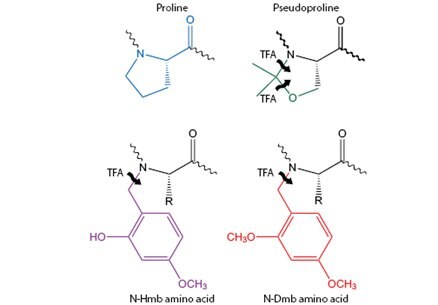
Figure 1.Secondary structure disrupting N-alkyl amino acids.

Figure 2.Designing a synthesis with secondary amino-acid surrogates. General guidelines for the use of secondary amino acid surrogates
- Optimal results are obtained if the amino acid surrogates are spaced 5-6 residues apart throughout the sequence.
- The optimum separation between an amino acid surrogate and a Pro residue is 5-6 amino acid residues.
- The minimum separation between an amino acid surrogate and another amino acid surrogate or Pro residue is 2 residues.
- Aim to insert an amino acid surrogate before regions of hydrophobic residues.
Pseudoproline Dipeptides
Mutter’s pseudoproline dipeptides are undoubtedly the most effective and simplest to use secondary amino acid surrogates, but are only appropriate for use in Cys, Ser- or Thr-containing sequences. They consist of a dipeptide in which the Cys residue or Ser/Thr residue has been reversibly protected as a proline-like TFA-labile thiazolidine or oxazolidine, respectively. The reason the pseudoproline residue is introduced as a dipeptide is because it avoids the need to acylate the hindered oxazolidine nitrogen. This has the added advantage of extending the peptide chain by two residues in one step.
These dipeptides are extremely easy to use: simply substitute a Cys, Ser or Thr residue together with the preceding amino acid residue in the peptide sequence with the appropriate pseudoproline dipeptide (Figure 3). The native sequence is regenerated on cleavage and deprotection. To achieve the maximum benefits, it is important to follow the guidelines set-out above.
Positioning of pseudoproline residues at regular intervals has proven to be an extremely effective approach for the synthesis of long and amyloidogenic peptides. This approach has been exemplified by the synthesis of a 95 residue peptide in remarkable purity through the expeditious use of 7 pseudoproline dipeptides in the synthesis of 101mer related to d2 domain of VEGF receptor, and parallel production of ubiquitin analogs.
Pseudoproline dipeptides can be introduced in the same manner as other amino acid derivatives. Pseudoproline dipeptide have been coupled using phosphonium and aminium activation reagents, such as PyBOP®, HBTU, TBTU, and HATU activation methods (Method 1a), as well as with carbodiimide-mediated coupling strategies, such as DIPCDI/HOBt (Method 3-15a).
On automated instruments, the simplest approach is to use the same amount of pseudoproline dipeptide as any other amino acid, since this avoids having to reprogram coupling cycles or make any manual intervention to the synthesis. Program the instrument to add a Cys, Ser or Thr residue, and remember to omit from the synthesis program the cycle for the next amino acid as this will be introduced as part of the pseudoproline dipeptide.
Using 5-fold excess of phosphonium- or aminium-activated pseudoproline dipeptide to resin functionality, coupling reactions are generally complete in 1 h. Lower excesses of reagent can also be used, but it then becomes advisable to check the completeness of reactions using an amine test, such as the Kaiser or TNBS test.
Regeneration of cysteine, serine or threonine from the pseudoproline occurs during the course of the TFA cleavage reaction using standard cleavage cocktails, such as TFA/water/TIS (95:2.5:2.5), and is generally complete in 3h.
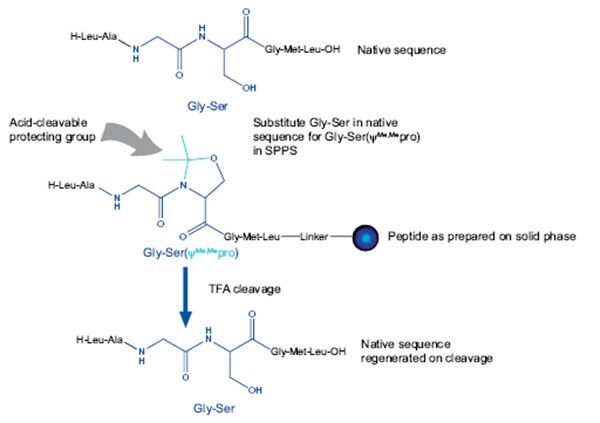
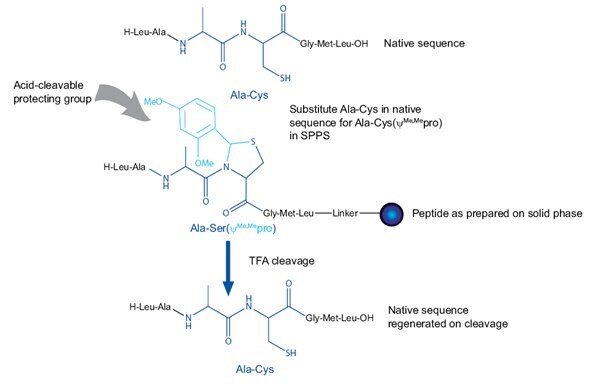
Figure 3.Relationship between peptide sequence and pseudoproline dipeptide.
Protocols for the Use of Pseudoproline Dipeptides
Method 1a: Manual coupling of pseudoproline & Dmb dipeptides
Phosphonium/aminium activation
- Dissolve derivative (5 eq.) and coupling reagent (PyBOP®, TBTU, HBTU, HCTU, or HATU, 5 eq.) in minimum volume of DMF or NMP.
- Add DIPEA (10 eq.) and mix thoroughly.
- Add immediately to Fmoc-deprotected peptide resin, and agitate for 1-2 h. Check completion of coupling by the TNBS test. If reaction is not complete, extent coupling time or repeat reaction using fresh reagents.
DIPCDI/HOBt activation
- Dissolve derivative (3 eq.) and HOBt (3 eq.) in minimum volume of DMF/DCM (2:1).
- Add DIPCDI (3 eq.) and mix thoroughly.
- Leave to activate for 10 minutes and then add to Fmoc-deprotected peptide resin, and agitate for 1-2 h. Check completion of coupling by the TNBS test. If reaction is not complete, extend coupling time or repeat reaction using fresh reagents.
Method 1b: Automated coupling of Pseudoproline dipeptides
Instruments using dry Fmoc-amino acids in cartridges
- Pack empty vials or cartridges with the appropriate amount of pseudoproline or Dmb dipeptide for the instrument protocols (i.e., 1 mmol for ABi 433 and 0.8 mmol for Pioneer).
- Program instrument to couple dipeptide as Ser, Thr or Gly residue (1 h coupling, HBTU, HATU activation). Omit amino acid cycle for next amino acid.
- Smaller excesses of dipeptides can be used, but the delivery volumes of coupling and base reagents will need to be modified in the coupling protocols to maintain the correct ratio of dipeptide/coupling reagent/base (1:1:2). Alternatively, the instrument can be programmed to pause after the wash step following Fmoc removal, and the dipeptide coupled manually by adding a solution of activated derivative to the reaction vessel.
Instruments that needle dispense solutions of reagents from vials (ACT 396, Zinsser 350)
- Dissolve derivative in DMF or NMP to exactly the same concentration as standard Fmoc-amino acid derivatives in reagent vial.
- Place vial containing dipeptide in an appropriate position of autosampler rack.
- Program instrument to couple dipeptide as Ser, Thr or Gly residue (1 h coupling, HBTU, HATU activation). Omit amino acid cycle for next amino acid.
Instruments that use stock solutions of Fmoc-amino acids with dedicated solvent lines (Protein Technologies Symphony)
While it is perfectly feasible to use pre-dissolved solutions of pseudoproline dipeptides on instruments such as the Symphony, this can be quite wasteful as reagent solvent lines would need to be primed with dipeptide solution before use. For such instruments , the cycle should be programmed to pause after the wash step following Fmoc removal and the “add” amino acid and coupling reagent steps in the cycle replaced by a “none” reagent step. The dipeptide can then be coupled manually by adding a solution of activated derivative to the reaction vessel.
Dmb Dipeptides
Dmb dipeptides work in exactly the same way and offer many of the same benefits as pseudoproline dipeptides but for Gly-containing sequences i.e. faster and more predictable acylation reactions, higher yields and purities of crude products, and less failed syntheses. Like pseudoproline dipeptides, insertion of a Dmb dipeptide introduces two amino acids at once and avoids the difficult acylation of the (Dmb)Gly residue.
Using them is very straightforward, one simply substitutes a Gly residue together with the preceding amino acid residue in the peptide sequence with the appropriate dipeptide (Figure 4). The native sequence is regenerated on TFA-mediated cleavage and deprotection.
For best results when using Dmb dipeptides, it is best to follow the guidelines set out in Figure 2. Dmb dipeptides can be introduced using any standard coupling method.
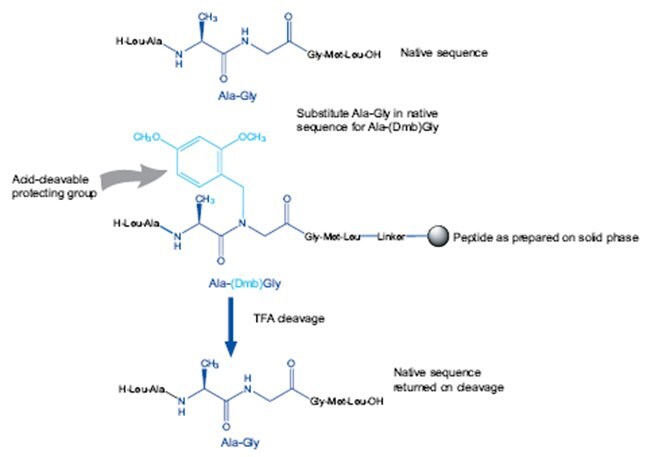
Figure 4.Principles of using Dmb dipeptides
Fmoc-Gly-(Dmb)Gly-OH is particularly useful for improving the synthesis of peptides containing the commonly occurring Gly-Gly motif. The production of such peptides is often problematic, owing to their propensity to aggregate and the difficulties in separating closely eluting desGly by-products. Insertion of Gly-(Dmb)Gly into a peptide sequence has the same benefits on synthetic efficiency as a pseudoproline dipeptide, preventing aggregation and improving acylation and deprotection kinetics. The use of this derivative was found to be essential for the synthesis of peptides related to nucleolin.
The Ala-Gly dipeptide sequence is frequently found in hydrophobic transmembrane and amyloidogenic peptides. Therefore, Fmoc-Ala-(Dmb)Gly-OH should prove to be a useful tools for the synthesis of such peptides.
For peptides containing the Asp-Gly motif, Fmoc-Asp(OtBu)-(Dmb)Gly-OH is highly recommended as its use for introduction of the Asp-Gly dipeptide completely prevents aspartimide formation.
Dmb/Hmb Amino Acids
Unlike the previously described pseudoproline and Dmb dipeptides, these derivatives are used to introduce backbone-amide protected residues individually rather than as dipeptides. However, once incorporated into a peptide they work in exactly the same way as pseudoproline and Dmb dipeptides by disrupting secondary structure formation, to give the same enhancements in synthetic efficiency.
By using such amino acid derivatives, one is not limited to introduction of backbone amide protection at either a Gly, Ser or Thr residue, as is the case with the dipeptide derivatives. However, the drawback to this approach is that it now becomes necessary to couple onto the hindered secondary amine of the Hmb or Dmb residue.
Sheppard and colleagues designed their Hmb derivatives to mitigate against this problem. Addition of the amino acid immediately following the Hmb-protected proceeds initially via internal base-catalyzed capture of the acyl component to give the phenyl ester which then slowly undergoes intramolecular O→N acyl transfer to afford the desired tertiary amide (Figure 5). Acylation of (Hmb)Gly is generally straightforward and works well for most amino acids with most coupling methods. For other Hmb residues, Sheppard and colleagues found that the use of PSAs in DCM or Fmoc N-carboxyanhydrides gave the best results. Other authors have also found TBTU/HOBt/DIPEA activation and amino acid fluorides to be effective. In practice, as the side-chain becomes more bulky, coupling gets more difficult and the steric hindrance of the incoming amino acid becomes an increasingly important factor. Using acid fluorides, the most difficult case, the addition of Fmoc-Val to (Hmb)Val, could be effected in a yield of 95% by carrying out the coupling overnight in toluene at 80 °C. Despite these limitations, Hmb derivatives have proven to be remarkably effective in a wide range of difficult and aggregated peptides.
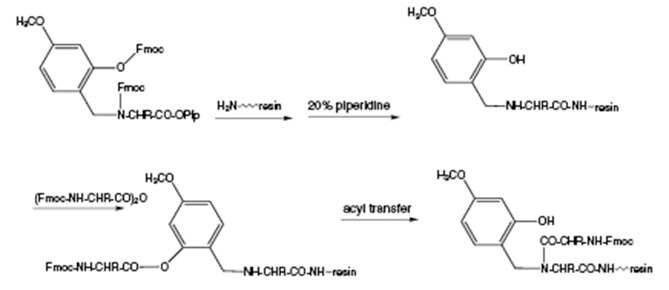
Figure 5.Mechanism of acylation of Hmb-protected residues.
(Hmb)Gly and (Dmb)Gly have particular applications in the synthesis of hydrophobic amyloid and transmembrane peptides. These sequences are extremely difficult to prepare using conventional methods, and are generally not amenable to pseudoproline substitution as they rarely contain multiple serine or threonine residues. Glycine, however does occur frequently in such peptides, often adjacent to hydrophobic residues such as Ile, Val, and Ala. Therefore, substitution of Gly by (Dmb)Gly or (Hmb)Gly should prove to be a highly effective method of overcoming these problems. Indeed, using six substitutions of the analogous (Tmob)Gly derivative, Bayer and colleagues were able to prepare a 64-residue transmembrane peptide in remarkable purity.
The coupling of Fmoc-(Dmb)Gly-OH can be achieved using standard methods such as PyBOP®/DIPEA. Following Fmoc removal with piperidine/DMF, the glycine secondary amine can be acylated with Fmoc-amino acids by a single coupling with PyBrOP® or HATU, or by using pre-formed amino acid fluorides. (Dmb)Gly is to be preferred over (Hmb)Gly in applications requiring post-synthesis acylation or phosphorylation as the Dmb group lacks potentially reactive hydroxyl functionality.
Deprotection
Dmb and Hmb groups are removed under the normal conditions required for the final cleavage and deprotection of the peptide. However, we recommend the addition of approximately 2% TIS to the cleavage mixture.
NOTE: the cleavage products of Dmb and Hmb can cause side-chain modification of unprotected Trp. We, therefore, strongly recommend the use of Fmoc-Trp(Boc) in all syntheses where these groups are used.
Solubilization of Aggregated Sequences
Acylation of the Hmb group with acetic anhydride in the presence of DIPEA greatly increases its acid stability, enabling peptides retaining Ac-Hmb protection to be generated directly on cleavage with TFA under normal conditions. Backbone protected peptides exhibit markedly improved solubility properties, facilitating purification by HPLC of otherwise intractable sequences. The free peptide may be regenerated, after deacetylation with 20% piperidine in DMF, by cleavage with TFA (Figure 6).
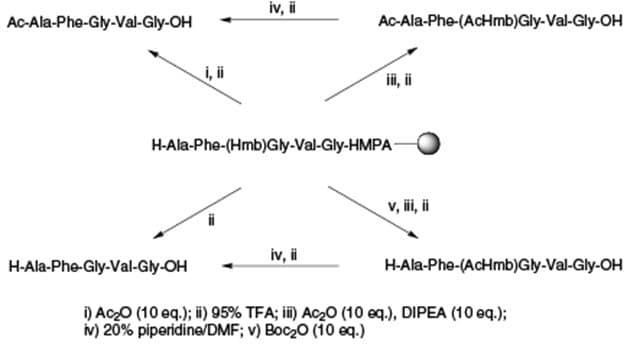
Figure 6.Synthesis of Ac-Hmb protected peptides.
References
To continue reading please sign in or create an account.
Don't Have An Account?