Lipopolysaccharides for Research – Structure, Function & Applications
Dr. Sai Sandeep Mannimala, Global Product Manager
MilliporeSigma
This article aims to explore the structure, function, and research applications of Lipopolysaccharides, the key component in Gram-negative bacteria. Discover the roles of LPS in bacterial defense, serological specificity, and its potential for vaccine development and drug research. To gain further insights into our range of products, we invite you to take a moment to complete this webform.
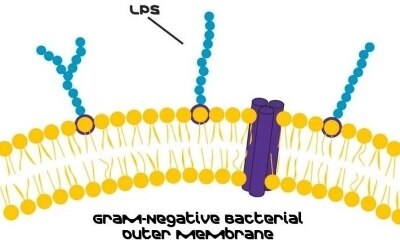
Introduction to Lipopolysaccharides
Lipopolysaccharides (LPS) are glycolipids and a major component of the outer membrane of Gram-negative bacteria. LPS is localized in the outer layer of the membrane and is, in non-capsulated strains, exposed on the cell surface. Primarily, they contribute to the integrity of the outer membrane and protect the cell against the action of bile salts and lipophilic antibiotics.1
Beyond its structural functions, LPS takes on a potent role as a bacterial endotoxin. Even in minute amounts, LPS can prove lethal upon entering the bloodstream.2 The body's compartmentalization typically prevents their entry, but factors like gut lesions or a lipid-rich diet can facilitate transport.3 LPS triggers a robust pyrogenic immune response and poses health risks in various products, particularly for individuals with gastrointestinal diseases.4
Additionally, LPS represents one of the conserved microbial structures responsible for activating the innate immune system. A compromised immune system intensifies LPS's endotoxic effects during infections, potentially leading to severe conditions such as septic shock.5 Gram-negative bacteria adapt by modifying LPS structure, linking LPS to autoimmune diseases and allergies. In the bloodstream, lipopolysaccharide-binding proteins act as carriers, transferring LPS to macrophages or serum lipoproteins. Macrophage-bound LPS induces proinflammatory responses, while lipoprotein delivery compromises the immune reaction and may lead to dyslipidemia.6
Structure and composition of Lipopolysaccharide
Lipopolysaccharides (LPS) have a complex molecular structure that's challenging to quantify in terms of molecular weight due to their heterogeneity and tendency to form aggregates of varying sizes. In its purest state, LPS are comprised of macromolecules with a molecular weight of 10-20 kDa. However, when exposed to treatment involving SDS and heat, the molecular weight of LPS typically falls within the range of 50 to 100 kDa. Under specific conditions, LPS can assemble into micelles of approximately 1,000 kDa without the need for surface-active agents, relying instead on divalent cation-sequestering agents like EDTA. When divalent cations such as Ca2+ and Mg2+ are present, LPS can adopt a bilayer structure that can pass through a 0.2 μm membrane but not a 0.025 μm membrane. In environments rich in divalent cations, LPS can even form vesicles up to 0.1 μm in diameter. The self-aggregation of LPS predominantly hinges on the lipid A component, which also enables it to adhere to hydrophobic surfaces. They consist of three main components:

Figure 1.General structure for bacterial lipopolysaccharides. Abbreviations: KDO: 3‑deoxy-α-D-mannooctulosonic acid; Hep: Heptulose (ketoheptose); NGa: Galactosamine; NGc: Glucosamine.
- O-Antigen: The outermost part of LPS, O-antigen, is a repeating oligosaccharide unit that varies between bacterial serotypes. It is the primary feature that distinguishes different bacterial strains, aiding in their identification.7
- Core Polysaccharide Chain: A hydrophilic core polysaccharide chain, which contains components like 3-deoxy-α-D-manno-octulosonic acid (KDO) and heptulose (ketoheptose). These parts are often phosphorylated or modified with phosphate-containing groups, contributing to the overall negative charge and structural stability.
- Lipid A: This is the hydrophobic section responsible for the toxic properties of LPS, which consists of glucosamine-based units with attached fatty acid esters.
It's important to note that LPS can exhibit structural variability, especially in the core and lipid A sections, leading to heterogeneity in LPS preparations.
Extraction and Purification
The preparation of LPS can be achieved using different methods, such as trichloroacetic acid (TCA),8 phenol,9 or phenol-chloroform-petroleum ether (PCP) extraction,10 with slight structural variations between them. TCA-extracted LPS and phenol-extracted LPS exhibit similar electrophoretic patterns and endotoxicity. However, the key differences lie in the amounts of nucleic acid and protein contamination. TCA extracts contain around 2% RNA and approximately 10% denatured proteins, whereas phenol extracts contain up to 60% RNA and less than 1% protein. Purification processes, including gel filtration chromatography and ion exchange chromatography, help reduce these contaminations, ultimately yielding LPS products with minimal protein and RNA content.
FITC (fluorescein isothiocyanate), TRITC (tetramethylrhodamine isothiocyanate), and TNP (trinitrophenyl) conjugates have been prepared by reacting LPS with either FITC, TRITC or 2,4,6-trinitrobenzenesulfonic acid, respectively.11 They are used in research on the T-independent B cell immune response to bacterial LPS.11
We offer Lipopolysaccharides with varying levels of protein and RNA, extracted using TCA, Phenol, and PCP methods, including those purified using Ion exchange and Gel filtration chromatography. Additionally, we also provide gamma-irradiated, detoxified, FITC-conjugate, and Ready-made solution alternatives to support a variety of research applications.
Our Lipopolysaccharide preparations have endotoxin levels of not less than 500,000 EU (endotoxin units) per milligram, unless otherwise noted. To put it in perspective, one nanogram of endotoxin equates to 0.5 EU in 1 ng of material, given an endotoxin level of 500,000 EU/mg (Limulus lysate assay) and 10 EU (chromogenic assay).
Functions
In Gram-negative bacteria, LPS play key role in shielding them from the effects of bile salts and lipophilic antibiotics.1 Further, LPS are stable against heat and associated with inducing a robust immune response in normal mammalian cells, particularly in the context of septic shock (septicemia) in humans.12 The lipid A component, within LPS, plays a pivotal role in its endotoxic activity. Studies have revealed that synthetic and natural-sourced Escherichia coli lipid A preparations produce identical bioactive outcomes, underlining the significance of lipid A in LPS.13 The primary receptor responsible for LPS recognition is the CD14/TLR4/MD2 receptor complex, which triggers the release of proinflammatory cytokines like tumor necrosis factor-α and interleukin-1.14 While lipid A predominantly activates immune responses, the polysaccharide component of Salmonella enterica LPS is also crucial for NF-κB activation.15
Research Applications
Lipopolysaccharides preparations serve as crucial tools for studying the intricacies of LPS structure,16 metabolism,17 immunology,18 cell biology,19 physiology,20 and toxicity,21 shedding light on the interaction between bacteria and the host immune system. Additionally, LPS stimulates the synthesis and secretion of key growth factors like interleukins, playing a pivotal role in various biological processes.22, 23.
Diagnostic Marker
Early Detection of Infection: LPS in the bloodstream serves as a sensitive marker for bacterial infections, allowing early diagnosis and intervention, ultimately improving patient outcomes.
Investigating Sepsis and Shock: LPS, particularly its endotoxin component, triggers inflammatory responses and severe conditions such as septicemia and shock, providing valuable insights for developing strategies in diagnosis and treatment.
Immunomodulation Studies
T-Independent B Cell Response: Conjugates of LPS with fluorescent molecules facilitate the study of the T-independent B cell immune response to bacterial LPS, contributing to a better understanding of immune system complexities.
Gut Microbiome and Immunity: LPS from different gut bacteria influences the immune system differently, such as promoting a healthy immune response or being linked to food allergies and autoimmune disorders, emphasizing the importance of understanding these interactions for optimal health.
Vaccines and Drug Delivery Research
Adjuvants: Low doses of LPS activate Toll-like receptors (TLRs) on immune cells, enhancing the effectiveness of vaccines and immunotherapies for stronger immune responses and potential therapeutic improvements.
Drug Delivery: LPS contributes to the development of targeted drug delivery systems, attaching therapeutic agents to LPS molecules for more effective and specific delivery, thereby reducing side effects.
Clinical Research
Developing Therapeutics: LPS plays a vital role in understanding and treating various diseases, including sepsis, autoimmune disorders, and cancer, with the aim of developing new therapies targeting specific pathways for improved patient outcomes.
Understanding Immune System: LPS serves as a valuable tool for investigating the intricacies of the immune system, aiding researchers in gaining a deeper understanding of how the body defends itself against pathogens and developing strategies to modulate immune responses for therapeutic purposes.
Storage and Stability
Solutions of Lipopolysaccharides LPS at 1 mg/mL in buffer or culture medium remain stable for around one month when stored at 2-8 °C. Frozen aliquots can be preserved for up to two years, but it's best to avoid repeated freeze/thaw cycles. To maintain LPS integrity, it's essential to store solutions in silanized containers, as LPS can adhere to plastics and certain types of glass, particularly when the concentration is below 0.1 mg/mL. If the LPS concentration exceeds 1 mg/mL, any adsorption to the vial's sides is minimal. If glass containers are used, ensure thorough mixing for at least 30 minutes to fully redissolve any adsorbed product.
References
To continue reading please sign in or create an account.
Don't Have An Account?