Virus-like Particle (VLP) Vaccine Bioprocessing and Formulation Steps
What are Virus-like Particles
A virus-like particle (VLP) is a biological nanoparticle that consists of the protective protein shell of a virus without its viral genome. VLPs are a specific class of viral subunit; they mimic the overall structure of virus particles but do not contain infectious genetic material. VLPs provide a high immunogenic response because of their high-density display of epitopes, the capacity to present multiple proteins to the immune system, and their size (typically around 40 nm; influenza VLP is 80–120 nm), which seems to be optimal for uptake by dendritic cells. Because VLPs don't contain viral genetic material and cannot replicate, they are noninfectious and safe. Furthermore, VLPs are unlikely to need adjuvants to be highly immunogenic.
Manufacturing of VLPs involves cell-based expression of the virus-shell protein. VLPs can be expressed in several heterologous expression systems. There are many VLP-based vaccines in commercial distribution and clinical trials that are produced in mammalian cell culture, baculovirus/ insect cell culture system, microbial fermentation (yeast, E. coli, etc.) and plants (tobacco, etc). VLPs are either assembled in vivo followed by purification from cell lysate, or the partially assembled protein is recovered from cell lysate and assembled into VLPs in vitro.
Generic VLP-based Vaccine Manufacturing Process
The manufacturing process for VLP-based vaccine is often complex. There are several methods of production. For the purpose of simplification, we will explain the production of baculovirus-mediated insect cell expressed VLPs. The downstream processing steps outlined in Figure 1 can be extrapolated to VLPs produced in other cell culture expression systems.
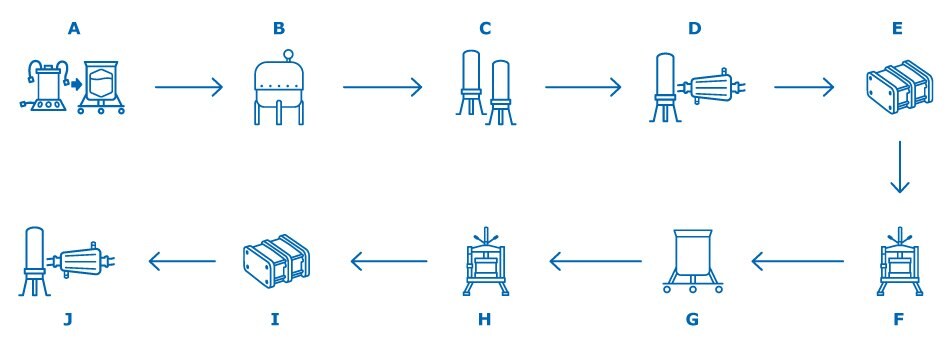
Figure 1. A general outline of the VLP-based vaccine manufacturing process. A: Media and Inoculum Preparation B: Cell Growth in Bioreactor / Virus Inoculation C: Clarification D: Bioburden Reduction E: Ultrafiltration / Diafiltration F: Purification Chromatography G: Baculovirus Inactivation or VLP Denaturation and Refolding H: Polishing Chromatography I: Ultrafiltration/Diafiltration J: Sterile Filtration or Final Filtration
Insect Cell Culture for the Production of VLPs
Generally, the insect cell line (Sf9, Hi-5) working cell bank is expanded through a series of shake flask cultures and transferred to a bioreactor at 1.0–1.2 x 106 cells/mL and allowed to expand until a defined culture volume at a cell density of 1.5 to 2.0 x 106 cells/mL is obtained. The culture is then infected with baculovirus at a multiplicity of infection (MOI) of 0.5–1.0. The cells are grown at 26–28 °C. VLPs are harvested approximately 48 to 96 hours post infection. The Sf9 cell density and viability at the time of harvest can be about 1.5 x 106 cells/mL with at least 20% viability. Some manufacturers harvest when the cell viability drops below 50%. Typically, culture is harvested by centrifugation at 1000 g for thirty minutes after which the fluid is decanted, and the cell paste stored at -60° to -80 °C.
Insect cells for the production of VLPs are mostly grown in commercially available serum-free insect media, a variation of IPL-41 basal medium. Serum-free insect cell media are supplemented with fetal bovine serum (FBS), protein hydrolysates, and a lipid/surfactant emulsion. Supplementation with heparin is also helpful to reduce the aggregation of cells.
According to a collaborative work carried out with Instituto de Biologia Experimental e Tecnológica (iBET), single-use bioreactors can be used for production of VLPs in insect cell culture. Typically, bioreactors have been used to produce hepatitis C VLP-based vaccine using Sf9 insect cells and Sf900II cell culture media. Agitation and sparging rates as well as inoculation at high cell density are key process parameters in a bioreactor that contributes toward more efficient VLP productivity. For more information on our new insect cell expression systems, the Sf-RVN® Platform, watch our “Novel Sf9 Rhabdovirus-negative (SF-RVN®) platform” webinar.
As an alternative, production of VLP in yeast is an attractive, low-cost alternative to insect and bacterial systems. S. cerevisiae batch cultures producing VLPs can be performed in a complete synthetic medium (CSM) with the addition of glucose (20 g/L) and yeast nitrogen base (6.7 g/L). For optimal performance, culture medium could be supplemented with leucine 1.8 mM, glutamate 20 mM, and succinate 50 mM. Potassium hydroxide (2N) is used for pH adjustments. The feeding medium for fed-batch cultures is 10 X CSM without supplements.
Cell Lysis to Release VLPs
VLPs are purified by resuspending cell paste in a tris buffer with 1.6 µm leupeptin. They are microfluidized to produce a cell lysate. Most VLPs are expressed in the cell and must be recovered by cell lysis, depending on whether the VLPs are secreted to the extracellular medium. There are reported cases of influenza VLPs produced in insect cell culture without cell lysis. In some cases, cell lysis is required to increase product yield.
Cell lysis releases host cell proteins (HCP) and host cell DNA (hcDNA) that must be removed. Sometimes Benzonase® endonuclease treatment is used to digest hcDNA, which is later removed by the downstream process. Therefore, the current trend is to design a clone compatible with an efficient secretory pathway.
Freeze-thaw, detergents, homogenization, or sonication are typically used for cell lysis, with high-pressure lysis being the most common. The harvest solution is forced through a small fixed orifice at a high pressure. The rapid transfer of the sample from a region of high pressure to one of low pressure causes cell disruption. Some manufacturers add NaCl to the medium to a concentration of about 0.4 to 1.0 M (preferably about 0.5 M) to avoid VLP aggregation. If chemicals are used, 1% Triton® X-100 solution is the most common detergent used. Sometimes 25 mM Na2HCO3, pH 8.3, is also used. In the process for Gardasil®4, the cell slurry is passed twice through a homogenizer to achieve a cellular disruption of greater than 95%. The mixture is incubated at 4 °C for 12 to 20 hours for complete lysis.
Chemically induced cell lysis can be carried out in appropriate mixing systems. Handling of buffers used in cell lysis and storage of lysate can be combined using a single-use bag, connector, and sampling system for an integrated operation.
Clarification of Cells and Cellular Debris from VLPs
Centrifugation, depth filtration, or microfiltration tangential flow filtration (TFF) can be used for clarifying the cells or cell lysate. Generally, microfiltration is preferred because it is robust and scalable. High shear during lysis micronizes the cellular debris. This, coupled with the large size of the VLPs, can make clarification problematic. The removal of cells and cellular debris from the cell culture medium containing VLPs is accomplished by either TFF, normal flow “dead-end” filtration (NFF), or centrifugation. Dead-end 0.2 µm filters work well for this application (yields >70%). In some cases, depth filters can be used, but proper optimization is required. Positive charge in depth filters can sometimes result in product loss due to adsorption. However, adding salt to the feed (100 mM NaCl) or performing pre- and post-buffer flushes with salt (0.5 M NaCl) can enhance the product recovery.
Nuclease Applications in VLP Processes
Benzonase® endonuclease is employed in the VLP purification process to degrade residual nucleic acids in order to meet regulatory purity requirements. The European Medicines Agency (EMA) and World Health Organization (WHO) allow 10 ng DNA per dose for parenteral vaccines and 100 µg DNA per dose for oral vaccines. Additionally, in order to minimize the risk of host cell nucleic acid oncogenicity, DNA size must be reduced to 100–200 base pairs in length.
Benzonase® endonuclease is typically applied as a batch incubation step that occurs either before or after lysate clarification. For adequate DNA digestion, 10–50 U/mL Benzonase® endonuclease is typically required, although the optimal Benzonase® endonuclease concentration varies with DNA and RNA concentration, incubation temperature, pH, time, and magnesium concentration. Maximum Benzonase® endonuclease activity occurs at 37 °C, pH 8. A concentration of 1–2 mM Mg2+ is essential to maintain Benzonase® endonuclease activity. Applying excess Benzonase® endonuclease under optimal conditions can result in sufficient nucleic acid digestion in one to four hours. In cases with reduced Benzonase® endonuclease concentration, or for processes that require incubation at low temperature or neutral pH, incubation times of 8 to 12 hours may be required. For each process, it is recommended that low-volume scouting experiments are performed to identify optimal Benzonase® endonuclease incubation conditions for adequate nucleic acid digestion. DNA digestion can be monitored with assays such as agarose gel electrophoresis, qPCR, or the threshold immunoassay.
After the incubation step, the Benzonase® endonuclease enzyme must be cleared in subsequent processing steps. In cases where Benzonase® endonuclease enzyme, a 60 kDa dimer, is sufficiently smaller than the VLP product, TFF can be used to achieve separation. Typically, a 300 kDa membrane is recommended to allow the passage of Benzonase® endonuclease while retaining the product molecules. For processes in which the molecular weight difference between VLP and Benzonase® endonuclease enzyme is not sufficient for TFF separation, either anion or cation exchange chromatography resins can be used. The Benzonase® endonuclease isoelectric point is 6.85. Benzonase® endonuclease removal can be monitored using an ELISA kit, which allows for specific quantification of residual active and nonactive endonuclease. DNA digestion using Benzonase® endonuclease enzyme can be carried out in a single-use mixer integrated with buffer bags and a sterile filter to introduce Benzonase® endonuclease enzyme and appropriate sampling system for quality control (QC) testing.
Concentration and Buffer Exchange
Depending on the type of VLPs, expression system, and VLP titer, an ultrafiltration/ diafiltration (UF/DF) step may be included in the process to concentrate and buffer exchange the product and make it ready for next step. Not every process requires this step. For example, the production of HPV L1 may not include this UF/DF step. Alternatively, influenza VLPs may require TFF with 300– 1000 kDa membranes. During concentration and buffer exchange, the HCPs are further reduced. Typically, the retentate is buffer exchanged with diafiltration (DF) to 25 mM Tris HCl, pH 8.0 for subsequent purification by ion-exchange chromatography.
Primary Purification of VLPs (Ion-Exchange Chromatography/Ultracentrifugation)
VLPs are commonly purified by ultracentrifugation over a CsCl, sucrose or iodixanol gradient. However, the use of CsCl for the purification step should be avoided. Indeed, in some cases, CsCl-purified VLP can appear to be heterogeneous in size because of broken particles and may introduce impurities into the downstream process. Aggregation during storage and functionality reduction can also cause complications in downstream process.
In sucrose ultracentrifugation, different and discontinuous concentrations of sucrose (20–60%) are layered. Each concentration is then collected for analysis after centrifugation. Given the differential density of each sucrose layer, the VLPs migrate until they reach a zone of similar density. This separates them from other contaminants that could not be previously removed. Typically, the discontinuous sucrose gradient is prepared in phosphate-buffered saline (PBS) buffer with 0.5 M NaCl, pH 7.2- or 20-mM Tris HCl, pH 7.5. This solution is then centrifuged either at 6,500 g for 18 hours at about 4–10 °C, or 37,000 g for three hours, or even 100,000 g for one hour at 4 °C. VLPs form a distinctive visible band between about 30% to 40% sucrose or at the interface (in a 20% and 60% step gradient) are collected from the gradient and stored. Sucrose can be removed by dialysis against PBS and diluted to 200 mM of NaCl in preparation for the next step in the purification process.
While ultracentrifugation techniques are well established and convenient for small-scale production, they can prove to be time consuming and poorly scalable. Alternatively, other purification methods such as ion-exchange chromatography can be used. Buffers that can be used in the ion exchange chromatographic step include phosphate (±citrate), Tris HCl, MOPS, HEPES, and sometimes stabilizing agents such as sucrose.
Weak ion-exchangers such as DEAE resins can be used to purify the VLPs. The material is first diluted with 20 mM Tris HCl to reduce salt concentration and then loaded in the column. After washing with Tris HCl buffer, the product is eluted with a phosphate buffer. In the final polishing step, the pH is adjusted. Alternatively, phosphate buffer at 20 mM with pH 7.5 and 150 mM NaCl has been used in diafiltration, column equilibration, and 1:2 dilution of the product prior to purification. Elution is performed using stepwise NaCl concentrations. Other anion-exchange resins have also been used— TMAE, DMAE, and Q resins—with significant reduction of DNA and endotoxin levels. These resins were tested using phosphate or HEPES buffer, pH 7.2 at varying NaCl concentration (150–1000 mM). Alternatively, TMAE resin can be loaded in 20 mM sodium phosphate buffer at pH 7. A pre-wash step with 20 mM sodium phosphate +0.4 M NaCl (to remove E. coli fragments) can be performed, and VLP elution is done at higher NaCl concentration. Rinsing with 1–2 M NaCl helps DNA removal. Prepacked columns containing ion exchange resins can be employed for this application.
In certain processes, membrane adsorption and monolith technology can provide better dynamic binding capacity (DBC) than particle-based resins. Although, in theory, monolith technology allows a straightforward scalability due to their flow-independent DBC, the actual scale-up of these columns can be challenging since their polymerization process is highly exothermic, which results in the possibility of an inhomogeneous structure. Multimodal resins that employ both size exclusion and binding-based separation also demonstrate VLP purification in flow-through mode. In this case, large molecular entities like VLPs are excluded from entering and interacting with the bead while small contaminants are trapped in the adsorptive core.
Baculovirus Inactivation
Insect cell–based expression systems can result in 1010-12 baculovirus particles in the process. As a regulatory requirement, baculovirus must be removed from the final product through orthogonal downstream steps during purification of VLPs. As a safety measure, some manufacturers perform inactivation of baculoviruses prior to removal. Inactivation is done by chemical methods using formalin or β-Propiolactone (BPL).
Selective precipitation and chromatographic methods (bind-and-elute or flow-through modes) can be used for removal and/or inactivation of intact baculovirus. Inactivation of baculovirus can be accomplished in multiple ways:
- Incubating in 0.2% BPL for three hours at about 25–27 °C.
- Incubating at 0.05% BPL at 4 °C for three days and then at 37 °C for one hour.
- Incubating in Triton® X-100 solution and tributyl phosphate (TBP) at concentrations of 1% and 0.3% respectively for thirty minutes at room temperature (25 °C).
The chromatography steps used for purification of VLPs are strictly monitored for their ability to separate VLPs from baculovirus. For example, ion-exchange chromatography can remove 102 to 105 baculovirus particles during purification of VLPs.
Polishing
When microbial systems are used, especially with E. coli, lipopolysaccharide (LPS) or endotoxins must be removed from the feed stream. Ion-exchange chromatography in bind-and-elute mode or membrane adsorption technology in flow-through mode works at this step. The hydrophobic nature of VLPs makes endotoxins interact with the particles themselves. This causes issues in the separation of LPS. Bound endotoxins can be released by treatment with solvents, mild detergents, or a combination of both. To avoid this problem, yeast (Pichia pastoris) or expression systems based on insect cells (Sf9) are preferred.
Weak ion-exchange chromatography resins have been used successfully for final polishing steps. In this step, VLPs pass through the column while residual baculovirus and DNA binds to the column. The flow-through fractions contain VLPs. However, the VLPs and some VLP-derived impurities can have similar electrostatic properties. When the difference in size is significant, one or more size-exclusion chromatography (SEC) steps may work as an alternative. Stabilizing agents and compounds prevent ion interactions between SEC resin and VLPs. For example, a Sucrose-Phosphate-Glutamic Acid (SPG) buffer made of 0.218 M sucrose, 0.0038 M KH2PO4, 0.0072 M KH2PO4, and 0.0049 K-glutamate, pH 8.0±0.2 may result in good VLP recovery when used with size-exclusion resin. It should be noted that UFDF constitutes an interesting option at this stage to remove lower-molecular-weight impurities. In addition, UFDF and SEC both efficiently allow buffer exchange for the final formulation with superior and easier scalability for tangential flow filtration.
Sterile Filtration and Formulation of VLP-based Vaccines
VLP-based vaccines are typically formulated in sucrose and Tween® detergent. Some final formulations may contain amino acids, amorphous aluminum hydroxyl phosphate sulfate, carbohydrates, L-histidine, mineral salts, polysorbate 80, sodium borate, etc. The final product is sterile filtered using a 0.22 µm filter.
Formulation of VLP-based vaccine can be achieved using single-use components. Individual components can be prepared in solution and filter sterilized to a 2D or 3D bag using 0.22 µm sterilizing grade filter. Mixing of different antigens, excipients (buffering agents, preservatives, stabilizers, detergents, etc.) and adjuvant can be done in a closed operation. Single-use bags containing formulation reagents can be connected to any mixer through sterile quick-connects. After compounding and formulation, the product can be aseptically transferred to single-use filling systems for final filling and vialing.
Summary
VLPs have gained continued interest because of their advantages over traditional vaccines. The absence of viral genomic material enhances safety of VLP-based vaccines. The choice of production platforms described in this section depends on several factors including cost and the need for post-translational modifications (PTMs), which can be essential in generating an optimal immune response.
Find more resources for virus-like particle vaccine manufacturing
References
To continue reading please sign in or create an account.
Don't Have An Account?